Characteristics of Electromagnetic Phenomena
1. Static may be only
solitarily, not oscillating electric and magnetic fields (MF). All fields are vectorial units (have a x, y, z component).
• Electric field E = U/d (unit: V/m)
• Magnetic field H = I/d (unit: A/m)
• Magnetic Induction B = H ·m0 ·mr (unit: 1 T (Tesla) = 104 G)
All magnetic field lines must close
themselves (equation: div B = 0)
2. Electromagnetic Radiation consists of rightangular orientated vectors of electric and magnetic field strengths
oscillating with frequency f. They spread out with the speed of light c ,have
the wavelength l = c/f and the Energy E=h·f .
• "Non ionizing" means: Generating no charge separation on atomic level
• "Non thermal" means: Absorbance causes no warming up, energy below the thermodynamical equilibrium.
3. Frequency range of EMF:
ULF (ultra low freq. <1 Hz)
ELF (extremely low
freq. 1 -300 Hz)
VLF (very low freq. 0.3-100 KHz) VHF (very high freq. 100-500 KHz)
UHF (ultra high freq.
>500-1000 MHz) EHF (extremely high
freq. >1 GHz)
Also Radio band assignments are used
(NF, HF, LW, MW, KW, UKW)
Man made Sources of
MF and EMF
• Power-lines and -consumers (16.33, 50, 60 Hz ELF).
• Cable communications (e.g. Telephone, Computer networks ELF-VHF).
• Radio communication, medicine, navigation and positioning (e.g.
Broadcast, TV, Tomograhy,
Radio-telephony, Cellular telephones (900 MHz-2.1 GHz),
Radar VHF-EHF).
Natural Sources of MF and EMF
Local variations of the geomagnetic field
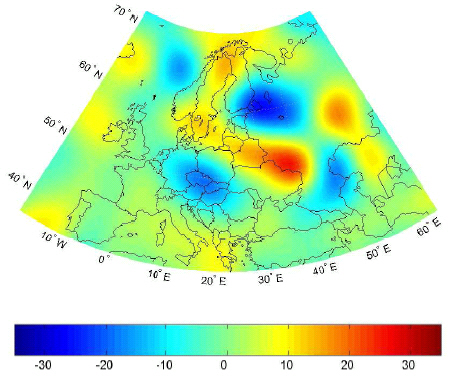
One important factor for such charts is the used kind of compensation
of long range geometry of the geomagnetic field. On this magnetic
anomaly chart of Europe the "Kursk magnetic anomaly", caused by a large
iron ore deposit in central Russia is well presented (intensive red
spot). Scale values are in nT. (source: T.Maier, Diss., University of
Kaiserslautern, Germany 2003). |
• Geomagnetic field
(static ~0.4 G with
• ULF-ELF variations).
• ELF Radiowaves from
meteorological Interactions in the lower
atmosphere.
• VLF to UHF Radiowaves
from ionization processes in the upper
atmosphere by cosmic
radiation.
(Below) Typical long term outdoor ELF Spectrum 1-100 Hz, the Schumann resonance's are visible, which originate as "standing waves" by the
reflexion properties of the earth surface and the Ionosphere and
superimposition after each turn. They are mainly supplied by NF- and
radio wave events in the atmosphere e.g. the "Spherics" of atmospheric
discharge processes.
|
|
Fig.2: High energetic charged particles, which periodically
increase during the 11 year solar sunspot cycle impact the geomagnetic
field on the sun side, and stretch it on the counterside. This effect
is modulated by the particle stream. (image source: NASA) |
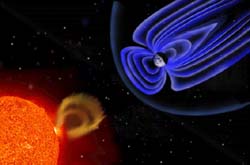 |
Fig.3: Around the geomagnetic poles the geomagnetic shielding effect is weak
and high energetic charged particles may penetrate to lower atmosphere
layers. Then the thinned gas in some 10 km altitude will be ionized and
emit light during recombination like a discharge lamp: auroras (polar
lights) originate, which can again can produce electromagnetic noise in
the radio frequency range. This again can feed back to the global
atmospherical Schumann resonance's. (image source: Mikel Bauer,
http://www.sonnenwinde.de) |
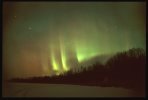 |
Wavelength and frequency
Any wave—including an electromagnetic wave—can be described by two properties: its wavelength and frequency. The wavelength of a wave is the distance between two successive identical parts of the wave, as between two wave peaks or crests. The Greek letter lambda (λ) is often used to represent wavelength. Wavelength is measured in various units, depending on the kind of wave being discussed. For visible light, for example, wavelength is often expressed in nanometers (billionths of a meter); for radio waves, wavelengths are usually expressed in centimeters or meters.
Frequency is the rate at which waves pass a given point. The frequency of an X-ray beam, for example, might be expressed as 1018 hertz. The term hertz (abbreviation: Hz) is a measure of the number of waves that pass a given point per second of time. If you could watch the X-ray beam from some given position, you would see 1,000,000,000,000,000,000 (that is, 1018) wave crests pass you every second.
For every electromagnetic wave, the product of the wavelength and frequency equals a constant, the speed of light (c). In other words, λ · f = c. This equation shows that wavelength and frequency have a reciprocal relationship to each other. As one increases, the other must decrease. Gamma rays, for example, have very small wavelengths and very large frequencies. Radio waves, by contrast, have large wavelengths and very small frequencies.
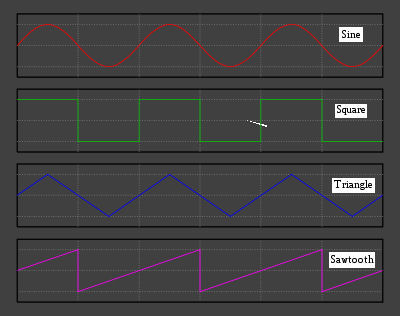 |
Common periodic waveforms include (t is time):
- Sine wave: sin (2 π t). The amplitude of the waveform follows a trigonometric sine function with respect to time.
- Square wave: saw(t) − saw (t − duty). This waveform is commonly used to represent digital information. It is square wave of constant period contains odd harmonics that fall off at −6 dB/octave.
- Triangle wave: (t − 2 floor ((t + 1) /2)) (−1)floor ((t + 1) /2). This is the integral of the square wave. It contains odd harmonics that fall off at −12 dB/octave.
- Sawtooth wave: 2 (t − floor(t)) − 1. This looks like the teeth of a saw. Found often in time bases for display scanning. It is used as the starting point for subtractive synthesis, as a sawtooth wave of constant period contains odd and even harmonics that fall off at −6 dB/octave.
|
Other waveforms are often called composite waveforms and can often be described as a combination of a number of sinusoidal waves or other basis functions added together.
The Fourier series describes the decomposition of periodic waveforms, such that any periodic waveform can be formed by the sum of a fundamental component and harmonic components. Finite-energy non-periodic waveforms can be analyzed into sinusoids by the Fourier transform.
Electromagnetism and Life
There are many theoretical foundations for the interaction between electro-magnetism and life.
Delta is the frequency range up to 3 Hz. It tends to be the highest in amplitude and the slowest waves. It is seen normally in adults in slow wave sleep. It is also seen normally in babies. It may occur focally with subcortical lesions and in general distribution with diffuse lesions, metabolic encephalopathy hydrocephalus or deep midline lesions. It is usually most prominent frontally in adults (e.g. FIRDA - Frontal Intermittent Rhythmic Delta) and posteriorly in children e.g. OIRDA - Occipital Intermittent Rhythmic Delta).
Theta is the frequency range from 4 Hz to 7 Hz. Theta is seen normally in young children. It may be seen in drowsiness or arousal in older children and adults; it can also be seen in mediation. Excess theta for age represents abnormal activity.
Alpha is the frequency range from 8 Hz to 12 Hz. Hans Berger named the first rhythmic EEG activity he saw, the "alpha wave." This is activity in the 8-12 Hz range seen in the posterior regions of the head on both sides, being higher in amplitude on the dominant side. It is brought out by closing the eyes and by relaxation. It was noted to attenuate with eye opening or mental exertion. This activity is now referred to as "posterior basic rhythm," the "posterior dominant rhythm" or the "posterior alpha rhythm." The posterior basic rhythm is actually slower than 8 Hz in young children (therefore technically in the theta range). In addition to the posterior basic rhythm, there are two other normal alpha rhythms that are typically discussed: the mu rhythm and a temporal "third rhythm".
Mu rhythm is alpha-range activity that is seen over the sensorimotor cortex. It characteristically attenuates with movement of the contralateral arm (or mental imagery of movement of the contralateral arm).
Beta is the frequency range from 12 Hz to about 30 Hz. It is seen usually on both sides in symmetrical distribution and is most evident frontally. Low amplitude beta with multiple and varying frequencies is often associated with active, busy or anxious thinking and active concentration. Rhythmic beta with a dominant set of frequencies is associated with various pathologies and drug effects.
Gamma is the frequency range approximately 26–100 Hz. Because of the filtering properties of the skull and scalp, gamma rhythms can only be recorded from electrocorticography |
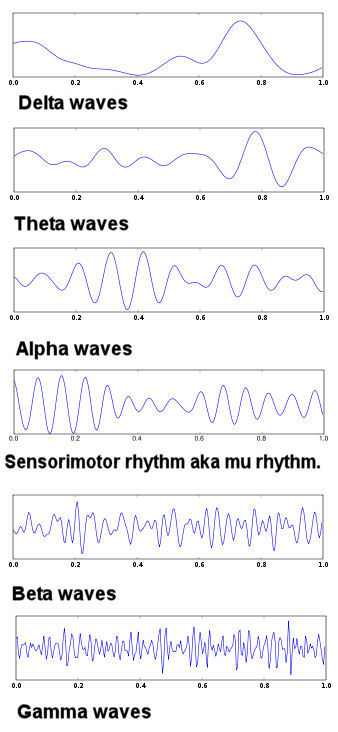 |
Behavior of electromagnetic fields
The behavior of the electromagnetic field can be resolved into four different parts of a loop: (1) the electric and magnetic fields are generated by electric charges, (2) the electric and magnetic fields interact only with each other, (3) the electric and magnetic fields produce forces on electric charges, (4) the electric charges move in space.
The feedback loop can be summarized in a list, including phenomena belonging to each part of the loop:
- charges generate fields
- the fields interact with each other
- fields act upon charges
- Lorentz force: force due to electromagnetic field
- electric force: same direction as electric field
- magnetic force: perpendicular both to magnetic field and to velocity of charge (
)
- charges move
Phenomena in the list are marked with a star ( ) if they consist of magnetic fields and moving charges which can be reduced by suitable Lorentz transformations to electric fields and static charges. This means that the magnetic field ends up being (conceptually) reduced to an appendage of the electric field, i.e. something which interacts with reality only indirectly through the electric field.
There are different mathematical ways of representing the electromagnetic field. The first one views the electric and magnetic fields as three-dimensional vector fields. These vector fields each have a value defined at every point of space and time and are thus often regarded as functions of the space and time coordinates. As such, they are often written as E (x, y, z, t) (electric field) and B(x,y,z,t) (magnetic field).
If only the electric field ( ) is non-zero, and is constant in time, the field is said to be an electrostatic field. Similarly, if only the magnetic field ( ) is non-zero and is constant in time, the field is said to be a magnetostatic field. However, if either the electric or magnetic field has a time-dependence, then both fields must be considered together as a coupled electromagnetic field using Maxwell's equations[1].
With the advent of special relativity, physical laws became susceptible to the formalism of tensors. Maxwell's equations can be written in tensor form, generally viewed by physicists as a more elegant means of expressing physical laws.
The behaviour of electric and magnetic fields, whether in cases of electrostatics, magnetostatics, or electrodynamics (electromagnetic fields), is governed in a vacuum by Maxwell's equations. In the vector field formalism, these are:
- (Gauss' law), (Gauss' law for magnetism), (Faraday's law), (Ampère-Maxwell law)
The Lorentz force law governs the interaction of the electromagnetic field with charged matter.
Sources:
http://www.scienceclarified.com/
http://en.wikipedia.org/ |